Laser Diagnostics
Laser Diagnostics
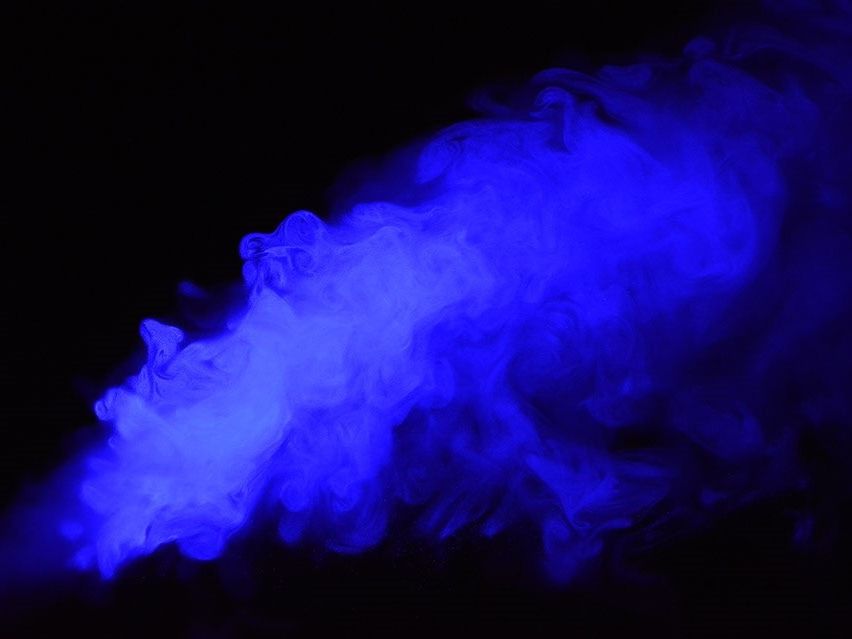
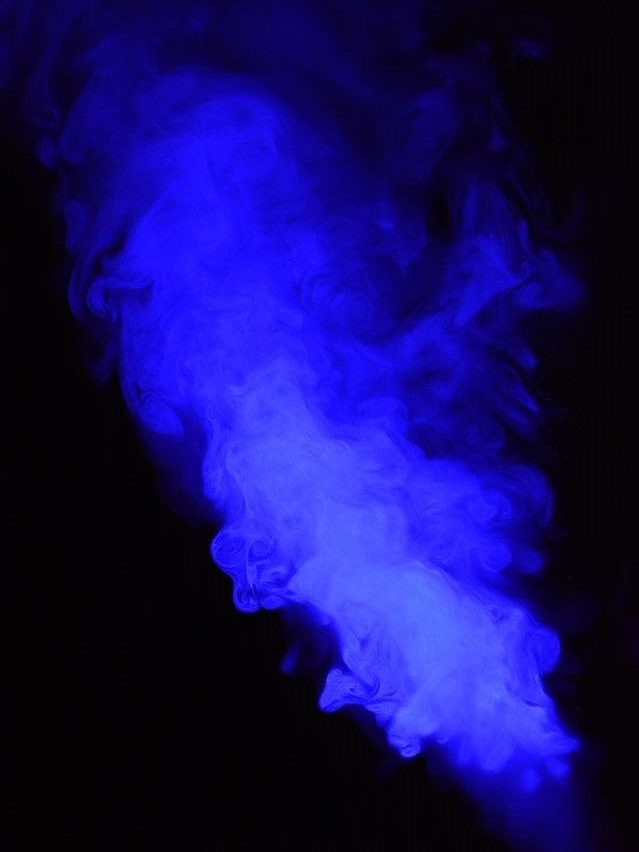
Laser diagnostics is a research area that studies how to measure the state of target material through light-matter interaction. Thanks to light’s unique characteristics, laser diagnostics delivers us information of inapproachable, and/or unsampleable targets, e.g. local equivalence ratio of supersonic combustors, distribution of short-lasting radicals in flames.
Among many kinds of light, laser diagnostics uses-obvious from its name-laser beams. Laser stands for light amplification by stimulated emission of radiation, and laser beam is generally characterized as monochromatic, coherent, and collimated, although there are some exceptions. These portraits not only facilitate experiments but also largely simplify theoretical analysis. Besides, the debut of pulsed lasers which emits intense laser beam within less than a few nanoseconds greatly broadened the scope of laser diagnostics by enabling us to exploit non-linear phenomena.
Specifically in combustion diagnostics, laser-induced breakdown spectroscopy(LIBS), laser absorption spectroscopy(LAS), and coherent anti-Stokes Raman spectroscopy(CARS) are commonly used to extract information on chemical component, temperature, and pressure, and shadowgraph, schlieren imaging, and interferogram are used for flow monitoring. Many other novel techniques are being developed as new types of systems are appearing.
In our laboratory, we study diagnosing techniques for scramjet combustors and high-pressure swirl flames.
Laser Absorption Spectroscopy (LAS)
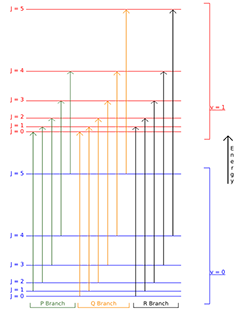
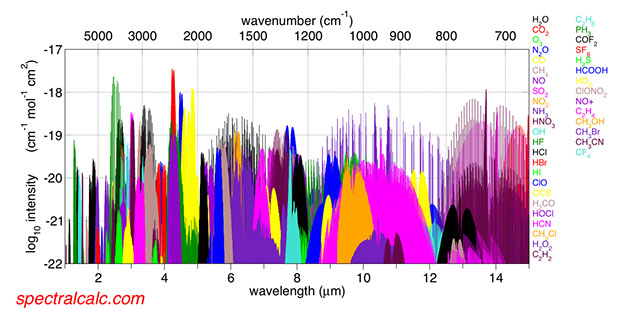
Tunable Diode Laser Absorption Spectroscopy (TDLAS) is one of the most quantitative techniques for measuring thermodynamic properties of molecules in gaseous phase. To date, TDLAS has been widely applied to combustion systems such as IC engines, gas turbines, scramjets, detonation combustors. Comparing other laser diagnostics, TDLAS is advantageous to arranging optical measurement systems to be affordable, compact, and adaptable. In a view point of quantum mechanics, TDLAS utilizes rotational and vibrational transition of energy states in gaseous molecules absorbing particular electromagnetic waves. This interaction only happens in the situation of the photon energy corresponding the difference of the two particular energy levels, hence this technique is available to identify such gases to be quantified in thermodynamic systems. Technically, the targeted gases equilibrating in the Maxwell-Boltzmann distribution are penetrated by tunable laser sources, then the absorbed lights are analyzed with the Beer-Lambert law. The absorption characteristics vary with thermodynamic conditions such as temperature, pressure or mole fractions of targeted species, or, conversely, such conditions are able to be quantified with analyzing the absorbed lights. There are several techniques to enhance the quantitativeness of TDLAS such as cavity-enhanced absorption, wavelength modulation, tomographic reconstruction. Research topics in RFL regarding the TDLAS include developing sensors measuring thermodynamic properties under harsh conditions for supersonic/hypersonic flows or high-pressure combustion applications.
Laser-Induced Breakdown Spectroscopy (LIBS)
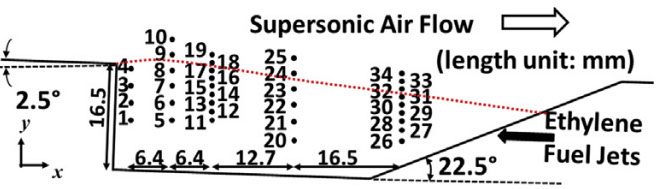
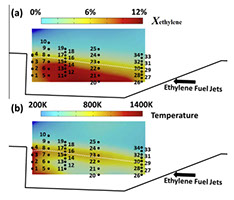
Laser-induced breakdown is used to measure density and concentration of gaseous mixtures. Above shows an example of LIBS in a cavity flameholder in a supersonic crossflow for measuring gas density and concentration of ethylene(fuel). The gas density was estimated using Plasma Energy(PE, energy absorbed by plasma) and the fuel concentration was calculated from emission spectra of plasma.
(H. Do et al., 2015)
Laser-Induced Plasma (LIP)
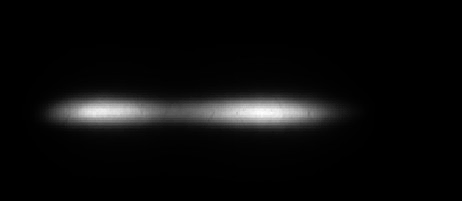
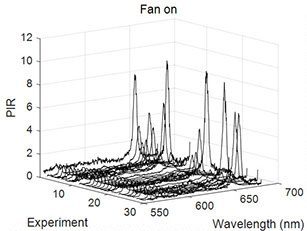
A focused laser beam with a sufficiently high irradiance (e.g., 100 GW/cm2 to atmosphere at standard condition) can generate a plasma volume. Commercial pulsed lasers like Nd:YAG laser with few nanoseconds pulse width instantaneously offer enough photon energy exceeding breakdown threshold. Before a laser pulse arrives a focal point (thus, irradiance is still less than the threshold), interaction between the photons and the gas molecules on a laser pathway occurs hardly. Therefore, we can employ this laser-induced plasma (LIP) as a sort of mechanical equipment with the minimal intrusiveness. For example, a LIP can be an optical igniter in a combustor, because it actively absorbs photon energy via multiphoton ionization followed by the inverse-Bremsstrahlung process and undergoes abrupt temperature increase up to approximately 60,000 K which is high enough to initiate chemical reactions. It even can be used as a measurement probe. A LIP is consisted of neutral or ionized atoms originated from the gas molecules within a laser focal volume. We can estimate the gas properties including density or mole fractions by capturing the emitted photons from highly excited species.
Research topics in Reacting Flow Laboratory include i) improving measurement accuracy with LIPs especially in harsh conditions like high pressure and temperature or supersonic flows, ii) understanding underlying physics in plasma formation, and iii) developing novel and effective diagnostic methods for practical applications. We utilize variety of optical equipment such as solid-state/gas/fiber/diode lasers, photodiodes, photo-multipliers, intensified CCDs, spectrometers, and other optics. With those, many different optical observing techniques are fully operational, e.g., spectroscopy, imaging, and photometry.